Felix Castellano
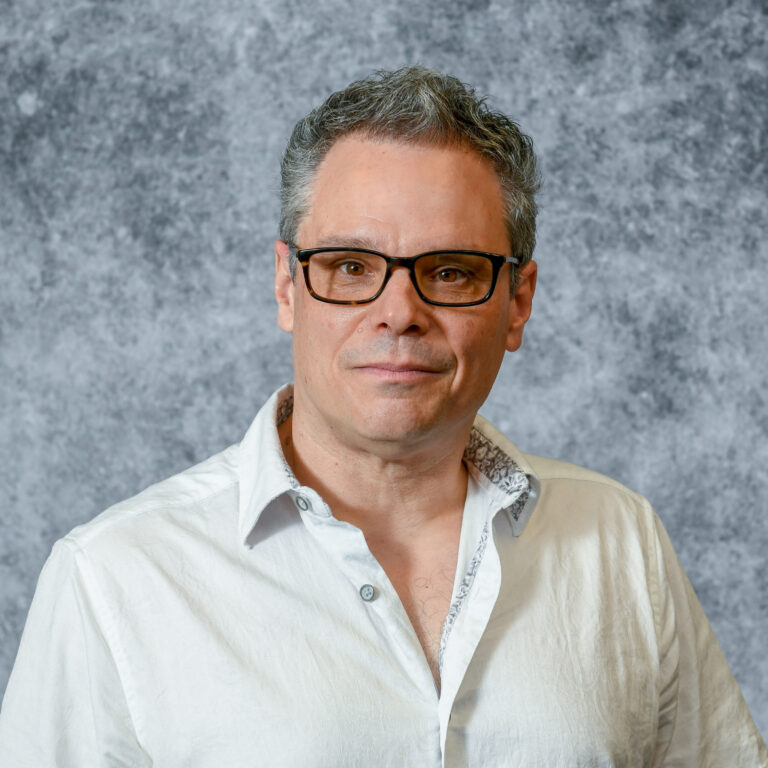
He/Him/His
Goodnight Innovation Distinguished Chair and Professor
Goodnight Innovation Distinguished Chair and Professor
Department of Chemistry
Partners Building III 319
Bio
Felix (Phil) Castellano earned a B.A. in Chemistry from Clark University in 1991 and a Ph.D. in Chemistry from Johns Hopkins University under the direction of Jerry Meyer in 1996. Following an NIH Postdoctoral Fellowship at the University of Maryland, School of Medicine, working with Joe Lakowicz, he accepted a position as Assistant Professor at Bowling Green State University in 1998. He was promoted to Associate Professor in 2004, to Professor in 2006, and was appointed Director of the Center for Photochemical Sciences in 2011. In 2013, he moved his research program to North Carolina State University where he is currently the Goodnight Innovation Distinguished Chair. He was appointed as a Fellow of the Royal Society of Chemistry (FRSC) in 2015, earned the I-APS Award in Photochemistry in 2019, and was elected as an AAAS Fellow in 2020. He is also the inaugural Editor-in-Chief of Chemical Physics Reviews, a peer-reviewed journal from AIPP. His current research focuses on metal-organic chromophore photophysics and energy transfer, photochemical upconversion phenomena, thermally-activated delayed photoluminescence processes, solar fuels photocatalysis, energy transduction at semiconductor/molecular interfaces, photoredox catalysis, fuel-forming chemical reactions, ultrafast transient bond-making and bond-breaking processes, and excited state electron transfer.
Education
Ph.D. Chemistry Johns Hopkins University 1996
B.A. Chemistry Clark University 1991
Publications
- Photo-Induced Bandgap Engineering of Metal Halide Perovskite Quantum Dots In Flow , ADVANCED MATERIALS (2025)
- Photo-ejected ligands hyperpolarized by parahydrogen in reversible exchange , CHEMICAL COMMUNICATIONS (2025)
- Photophysical Characterization and Excited State Dynamics of Decamethylruthenocenium , JOURNAL OF PHYSICAL CHEMISTRY A (2025)
- Photophysical and Time-resolved Infrared Properties of Long-Lived Rhenium(I) 4,5-Diazafluorene Tricarbonyl Chromophores , CHEMPHYSCHEM (2025)
- Chemical Physics Reviews as a platform for the chemical physics community: The first four years , CHEMICAL PHYSICS REVIEWS (2024)
- Color morphing surfaces with effective chemical shielding , NATURE COMMUNICATIONS (2024)
- Correction to “Steric and Electronic Influence of Excited-State Decay in Cu(I) MLCT Chromophores” , Accounts of Chemical Research (2024)
- Deciphering Charge Transfer Processes in Transition Metal Complexes from the Perspective of Ultrafast Electronic and Nuclear Motions , JOURNAL OF PHYSICAL CHEMISTRY LETTERS (2024)
- Direct Evidence for Buffer-Enhanced Proton-Coupled Electron Transfer in Metal Aquo Bond Formation , (2024)
- Enhanced Visible Light Absorption in Heteroleptic Cuprous Phenanthrolines , INORGANIC CHEMISTRY (2024)
Grants
Advanced spectroscopies with the state-of-the-art light sources provide unprecedented opportunities for capturing correlated electronic/nuclear motions in real time and mapping out excited state trajectories on the potential energy landscape. In particular, it is important to understand the impact of coherent electron and nuclear motions in energy and electron transfer processes that lead to chemical reactions, and how to control these motions to steer the reaction in desirable directions. The proposed work aims at directly measuring vibronic coherence in molecular systems and their effects in energy transfer processes in supramolecular systems or aggregates based on Pt(II) dimer molecules.
The proposed work selects transition metal complexes (TMCs) and their hybrids with inorganic nanoparticles (TMC/NP) as platforms to study effects of ultrafast coherent electronic or atomic motions in photochemical reactions to transfer energy and charges or to break or form bonds in chemical reactions. The project aims at understanding how ultrafast electronic and nuclear coherent motions in TMC and TMC/NP materials lead to selected outcomes in photochemical reactions, and how these chemical reactions can be controlled using external stimuli that couple with phases of the electronic and atomic motions. A key component of this program will be the direct detection of coherent nuclear and electronic motions correlated to light energy capture and conversion in TMC and TMC/NP systems using the femtosecond (fs) X-ray pulses from the X-ray free electron laser, Linac Coherent Light Source (LCLS). Both ultrafast laser and X-ray measurements will be used to track excited-state electronic and nuclear structural dynamics, coherent excited-states dynamics, and transitions from coherent excited to product states. Ultrafast X-ray studies at LCLS will be augmented with measurements from picosecond synchrotron X-ray slicing light sources to prototype LCLS experiments and characterize reaction outcomes using coherence phase relevant triggering schemes in TMC and TMC/NP materials. Theory will be partnered with experiments to achieve a fundamental understanding of excited-state electronic and nuclear coherences underlying photochemical reaction dynamics, and to elucidate and model spectral and dynamic features observed in the laser and X-ray experiments. The team includes experimentalists and theorists with the expertise ranging from synthesis, ultrafast laser spectroscopy, to forefront theoretical calculations to model coherent control and ultrafast photochemical reaction trajectories as well as X-ray absorption/emission spectroscopy and scattering. The cohesive approach of this project is organized by two themes: 1.) ultrafast electronic and structural coherence in TMC and TMC/NP materials relevant to solar energy conversion and catalysis, 2.) coherent control of electron/energy transfer in transition metal complex/nanoparticle interfacial energy/electron transfer processes on the ultrafast time scales.
The mission of the Center for Hybrid Approaches in Solar Energy to Liquid Fuels (CHASE) is to evaluate molecule/materials hybrids for cooperative sunlight-driven generation of liquid fuels from CO2, H2O, and N2. The CHASE approach relies on fundamental mechanistic studies to develop and evaluate technological advances that harness the individual strengths of molecules and materials to cooperatively overcome longstanding challenges in the robust and selective conversion of abundant feed-stocks to liquid fuels and O2 driven by sunlight. This approach requires a diverse and deeply collaborative research Hub. Led by a core team at the University of North Carolina at Chapel Hill (UNC), CHASE features strategic satellite teams at proximal institutions. The team structure will provide scientific expertise and research infrastructure to: (a) synthesize new atomically and morphologically precise molecule/ materials hybrids for solar energy conversion, (b) address questions of unparalleled depth about structure, dynamics, and mechanism in complex hybrids, and (c) rapidly build on discoveries to expand basic energy science knowledge and prepare the next generation of solar fuels scientists. The fundamental challenges in liquid solar fuels generation are now clear, thanks in part to JCAP research. The CHASE approach, defined by precise molecule/materials hybrids and comprehensive mechanistic inquiry through sophisticated theory and advanced photophysical, photochemical, and catalysis studies, will provide ever-deeper understanding and design principles to overcome these challenges and make liquid solar fuels a reality.
We propose a quantum spin technology to image biochemical pathways in the rhizosphere with unprecedented chemical detail and sensitivity. Specifically, the proposed technology transfers the quantum entangled nuclear spin order of hydrogen gas, to metabolites, including nitrate, amino acids, nitrogen and pyruvate, to enable molecular imaging of their metabolic transformations without any penetration depth limitations such that molecular turnover and metabolism can be observed directly in soil.
The proposed research is composed of two parallel lines of inquiry addressing fundamental aspects of photo-induced energy transfer processes as they relate to the emerging topic of solar photon wavelength upconversion achieved through the regenerative photochemical process of sensitized triplet-triplet annihilation (TTA). The concepts developed in this proposal address topics and processes central to solar energy conversion while being cognizant of sustainable sensitizer design and compatibility of the proposed photochemical processes within aqueous environments. First, we seek to translate sensitized TTA-based upconversion into more sustainable formats using highly abundant elements in sensitizer design. Two representative molecular designs will be developed to promote intramolecular triplet state generation for use in upconversion schemes: (1) new CuI charge transfer sensitizers designed to possess markedly extended excited state lifetimes using pendant triplet acceptors to intramolecularly produce either ����������������pure��������������� organic triplets or equilibrated excited states based on the triplet reservoir effect; and (2) high extinction coefficient SnIV porphyrin sensitizers covalently tethered to energetically appropriate acceptors/annihilators in their axial positions, thereby circumventing the limitations imposed by bimolecular triplet sensitization. As a result, the typical sequence of two consecutive bimolecular reactions, triplet sensitization and annihilation, becomes reduced to only the latter which will then take place between two energized acceptor/annihilator molecules located on two distinct porphyrins. The second series of proposed investigations address a key criterion for potential integration into solar fuels producing schemes, namely, photochemical upconversion that decisively functions in water. In order to promote regenerative upconversion photochemistry in water: (1) we will directly address the problem by evaluating the photochemical interactions occurring between water-soluble phthalocyanine triplet sensitizer donors and acceptors/annihilators initially based on high fluorescence quantum efficiency perylenediimide (PDI) dyes, affording near-IR-tovisible light conversions; (2) micellar nanoreactors will be used to host/encapsulate various hydrophobic upconversion pairs in water, absorbing low energy light and radiating higher energy photons into the immediately adjacent aqueous solution; and (3) mesoporous silica nanoparticles will be used to examine upconversion occurring in nanoporous environments either between surface-anchored sensitizers and freely diffusing acceptors/annihilators or between chromophores loaded/adsorbed into the pores of the material. The nature of the micellar nanoreactors and mesoporous silica will permit detailed photophysical/photochemical examinations of these materials in water.